RF converters -- a technology enabling wideband radios
StorySeptember 18, 2019
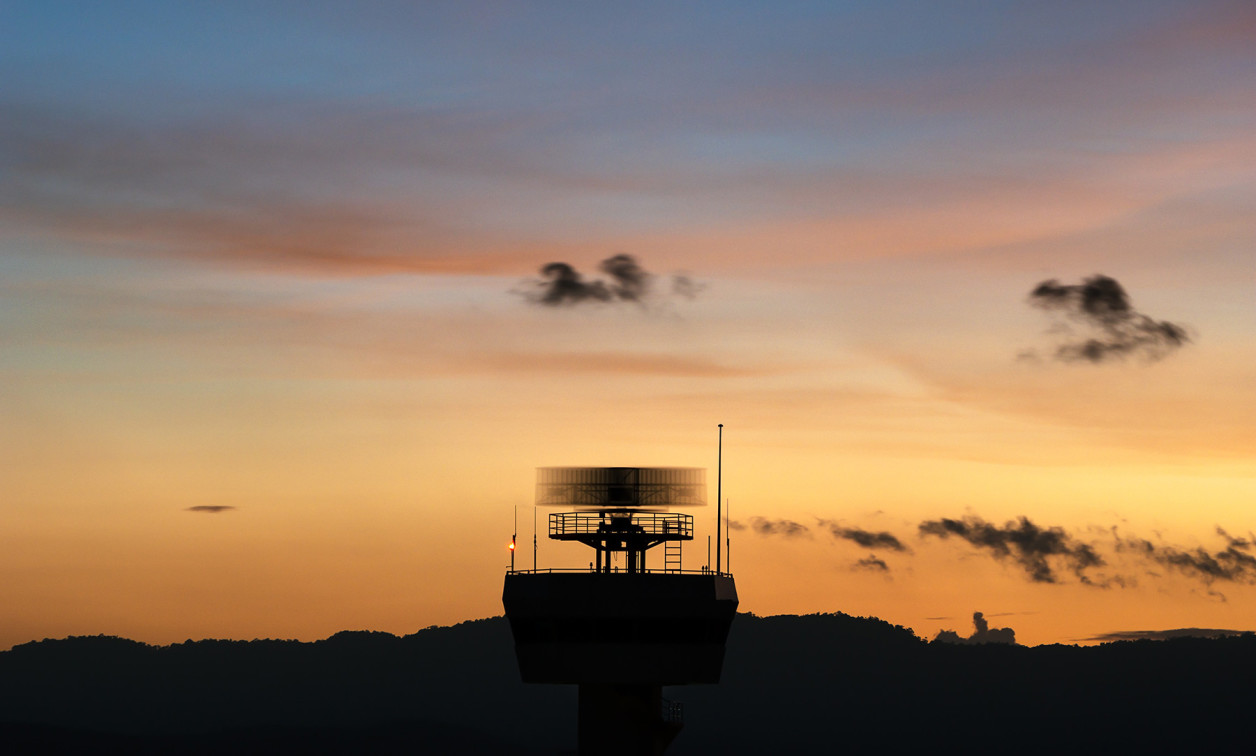
One design constraint that faces every military radio designer is the trade-off of designing for signal bandwidth with the highest possible quality versus the power consumption of the radio. The way in which the radio designer meets this constraint determines the size and weight of the radio and fundamentally influences the placement of the radio, which includes buildings, towers, poles, underground vehicles, packs, pockets, ears, or glasses.
Each radio location has an amount of power available that is commensurate with its location. A building or a tower, for example, will likely have more power available to it than a smartphone in a pocket or a Bluetooth headset in an ear.
As semiconductor companies have integrated more functionality and higher performance into the same- or smaller-size component, the equipment that uses these components has delivered on the promise of a radio that is smaller, more functional, lighter, or all three in some cases. These upgrades enable placement of the equipment in locations that were previously prohibited due to some other constraint, such as the amount of real estate needed for a building that is reduced when the unit can go on a tower, the size of a tower radio unit that can be reduced to a pole unit if the weight of the unit is low enough, or a unit that was required to be carried in a vehicle due to its weight that can now be carried in a pack.
Today’s environment is filled with legacy installations that require buildings, towers, poles, and vehicles. Driven by the need to connect the people in the world to each other, engineers meet that challenge by designing equipment with the available components at that time, delivered to us via the communications-rich environment we have today. People can talk, text, IM, photograph, download, upload, and browse nearly anywhere on one of several different networks, including mobile networks wireless LANs, ad hoc short-range wireless networks, and others. These all connect to the broadband wired network on which the data is carried by RF cables, and eventually by optical fiber. (Figure 1.)
Figure 1 | RF converters enable wideband radios that can service demanding data asks like streaming video and gaming.
|
Enhanced video experience
As several studies have shown,1, 2 the demand for data is projected to continue to increase well into the next decade. This mounting number is driven by a seemingly insatiable demand for richer data content that needs wider bandwidths. For example, cable television and fiber-to-the-home carriers continue to compete on broadband services to the home by offering higher-speed connections and more high-definition TV channels. The move to ultrahigh definition (UHD or 4K definition) TV calls for more than twice the capacity of HD TV and requires wider channel bandwidths than are being used today.
In addition, immersive video, which includes virtual reality (VR) – as well as gaming and 3D effects like 180-degree or panoramic viewing with multidimension freedom, all with 4K UHD TV – will demand as much as 1 gigabit of bandwidth per user.2 This ask goes well beyond the already demanding needs of simple 4K UHD TV broadcast and streaming. Moreover, online gaming requires symmetrical data bandwidths in the network since latency times are crucial, a need that is driving development of much wider-bandwidth upstream transmission capability. This need for wider upstream capability is, in turn, driving equipment makers to upgrade their designs to enable symmetrical, wide-bandwidth transmissions.
The enhanced capabilities of today’s RF converters are crucial to enabling advances in the delivery of such rich video content. They must be able to create high dynamic range signals with excellent spurious-free performance in order to enable the use of higher order modulation schemes such as 256-QAM [Quadrature Amplitude Modulation], 1024-QAM, and 4K-QAM. These higher-order modulation methods are needed to increase the spectral efficiency of each channel, since the installed coaxial cable plant and distribution amplifiers have a finite bandwidth of 1.2 GHz to 1.7 GHz. Higher performance in the head-end transmission equipment extends the usable life of the installed equipment base, easing capital budget constraints and allowing multiple service operators (MSOs) a longer window of time in which to upgrade their equipment and transmission systems.
Multiband, multimode test
Today’s smartphones resemble traditional mobile phones even less as more features are packed into them. Many of these features have radios associated with them; therefore, the mobile device of today has upward of five or seven or more radios in it. Because all of these radios must be tested when the smartphone is produced, makers of multimode communications testers face new challenges. There is a need for speed to keep test costs down, despite the number of tests increasing with the number of radios. Building different radio hardware for each radio in the mobile device becomes impractical with regards to size and cost of the tester. With more bands opening or being proposed for mobile services,3 the challenge of testing mounting numbers of radios in the mobile device increases.
This challenge can be addressed well by RF converters: In both the transmitter and receiver, RF converters can provide flexibility that cannot be achieved with conventional radios. Wideband RF converters enable testers to capture and directly synthesize signals in every band at the same time, enabling simultaneous test of multiple radios in the mobile device. With channelizers built into the RF digital-to-analog converter (DAC) and analog-to-digital converter (ADC), those multiple radio signals are efficiently processed in the converters. For example, in Figure 2, three channelizers per RF DAC are shown, enabling three different signals and bands to be directly synthesized, combined, and then upconverted digitally with the numerically controlled oscillator (NCO) before being converted to an RF signal by the RF DAC.
Figure 2 | Example of an RF DAC with channelizers.
|
Other market segments – such as testing equipment for defense and aerospace – are seeing increasing need for wideband test solutions for pulsed radar and military communications. Due to the number and types of radar, electronic intelligence, electronic warfare (EW) equipment, and communications equipment needing to be tested, the test-equipment manufacturer must create a flexible instrument with a rich set of features.4 For example, arbitrary waveform generators must be able to create various signals, including linear frequency modulation, pulsed signals, phase coherent signals, and modulated signals across a wide range of output frequencies and bandwidths.
Measurement equipment must be equally capable in order to receive such signals when testing the exciter or transmitter. RF converters handle this application by enabling direct RF synthesis and measurement at RF frequencies. In some cases, this approach can eliminate the need for an up- or down-conversion, and in other cases can reduce the number needed to a single conversion. It can also simplify the hardware and thus reduce its size, weight, and power (SWaP) requirements. The addition of digital features such as channelizers, interpolators, NCOs, and combiners makes for efficient signal processing on dedicated, low-power CMOS [complementary metal-oxide semiconductor] technology.
Software-defined radios
RF converters can be a critical enabler in software-defined radios. With the ability to directly synthesize and capture radio frequencies in the multi-GHz range, RF converters simplify the radio architecture by eliminating entire up- or down-conversion stages, instead implementing them digitally. The removal of the analog conversion stage and the associated mixers, LO [local oscillator] synthesizers, and filters reduces the SWaP of a radio, enabling the radio to be situated in more places and operate from smaller power supplies. Such technology makes it possible for the radios to be small and light enough to be hand-carried, driven in small ground vehicles, or mounted in various airborne assets such as planes, helicopters, and unmanned aerial vehicles (UAVs).5
In addition to enabling better communication across platforms, radio hardware built with RF converters has the potential to be multifunction, as well as multimode and multiband. Because RF converters are now able to reach to the lower radar bands, and in the near future will reach the higher bands, the concept of a single unit that can be used as both a radar and a tactical communications link can become a reality. Such a unit offers clear leverage in terms of field repairs, upgrades, and procurement procedures and costs.
The ability to directly synthesize and capture radar frequencies makes RF converters ideal for phased-array radar systems. Because direct RF converter synthesis and capture eliminates so much conventional radio hardware, an individual signal chain is much smaller and lighter. Thus, packing many of these radios into a smaller space is possible. Arrays suitable for ship-mounted or ground-based phased arrays, as well as smaller arrays and units for signal intelligence operations, can be built with reduced SWaP. (Figure 3.)
Figure 3 | Software-defined radio (SDR) powered by RF converters enables connected communications across platforms.
|
The technology behind RF converters
One of the key technology advancements that makes RF converters possible is the continuous march toward finer-line CMOS processes. As the gate length and feature size of the basic CMOS transistor becomes smaller, digital gates get faster, smaller, and lower-power.6 This setup enables significant digital signal processing (DSP) to be included on-chip with the RF converter with reasonable power and area. The inclusion of digital channelizers, modulators, and filters that are software-programmable are critical to constructing an efficient and flexible radio. This more efficient DSP also opens the door to use digital processing to help correct analog deficiencies in the converter. On the analog side, each new node provides faster transistors that have better matching per unit area. These improvements are critical for faster high-precision converters.
Process-technology advancement alone is not sufficient, however: Several key architectural advancements also make these converters possible. The architecture of choice for RF DACs is the current steering DAC architecture. The performance of this type of DAC is dependent on the matching of the current sources that comprise the DAC. Uncalibrated current source matching is proportional to the square root of the area of the current source.7 The matching per unit area will improve with each technology node. However, even in the most advanced nodes, a current source with low enough random mismatch for a high-resolution converter would be very large. Having such a large current source would make the converter large and – more critically – the parasitic capacitance of this large current source degrades the high-frequency performance of the DAC.
A much more attractive solution is to calibrate smaller current sources to achieve the desired level of matching, which can significantly reduce the added parasites from the current source and thus enable the desired linearity performance without compromising the high-frequency performance. If done correctly, this calibration can be made very stable across temperature, which means the calibration need only be done once. Stable one-time calibration avoids periodic background calibration, which saves operating power and alleviates concerns about spurious products being created due to the calibration running in the background.8
Another architectural choice that helps meet the desired converter performance metrics at very high speeds is the choice of switch architecture used to steer the DAC current. The traditional dual-switch structure (Figure 4) has several drawbacks when operated at very high speeds.9, 10 Since the data driven into the dual switch can stay the same anywhere from one to many clock cycles, the tail node will have a data-dependent amount of time to settle. If the clock rate is slow enough for this node to settle within one clock period, this is not a problem. However, at very high rates this node will not settle fully in one clock period and thus the data-dependent settling time will cause distortion in DAC output. If a quad switch (Figure 5) is used, the data signals are all returned to zero. This leads to the tail node voltage being independent of data input, which alleviates the problem mentioned above. The quad switch also enables the DAC data to be updated on both edges of the clock. This feature can be used to effectively double the DAC sample rate without doubling the clock frequency.11
Figure 4 | The traditional dual-switch architecture used to steer the DAC current.
|
Using a well-designed current source calibration algorithm and a quad switch current steering cell – in combination with today’s fine line CMOS processes – enables design of a DAC that can sample at very high rates with excellent dynamic range, which allows for synthesis of high-quality signals across a wide range of frequency. When this wideband DAC is combined with supporting DSP, it becomes a very flexible high-performance radio transmitter that can be configured to provide signals for all of the different applications mentioned previously in this article.12
Figure 5 | A quad-switch structure can alleviate many of the problems encountered by dual switch structures when operated at very high speeds.
|
Future radios
While the RF converters of today are already enabling radical changes in radio architecture design, they are poised to enable even greater changes in the future. As process technology continues to advance and RF converter design is further optimized, the RF converter’s impact on power consumption and size of a radio will continue to shrink. These opportune technology advances come just in time to enable the next generation of radios, such as emerging 5G wireless base station applications, as well as large-scale phased-array radars and beamforming applications. Deep-submicron lithography will enable even larger amounts of digital circuitry to be placed on the RF converter die, integrating critical compute-heavy functions like digital predistortion (DPD)13 and crest factor reduction (CFR) algorithms that help to improve power amplifier efficiency and reduce overall system power dramatically.
Such integrations will relieve the pressure on power-hungry FPGA [field-programmable gate array] logic and instead move those functions to power-stingy dedicated logic. Other possibilities include integrating the RF converter and its digital engines with RF, microwave, or millimeter-wave analog components, further reducing size and further simplifying the radio design, and delivering a bits-to-antenna, system-level approach to radio design.
References
1. “5G Radio Access.” Ericsson, April 2016.
2. “Consumer Survey Report on Typical Future Mobile Applications.” Huawei Wireless X Labs.
3. “Notice of Inquiry FCC 17-104.” Federal Communications Commission, August 2017.
4. John Hansen. “Radar, Electronic Warfare, and Electronic Intelligence Testing.” Agilent Technologies, August 2012.
5. Henry S. Kenyon. “New Radios, Waveforms Move Military Communications into the Sky.” Signal, October 2013.
6. William Holt. “Moore’s Law: A Path Going Forward.” 2016 IEEE International Solid-State Circuits Conference, IEEE, 2016.
7. A.C.J. Duimaijer, Anton Welbers, and Marcel Pelgrom. “Matching Properties of MOS Transistors.” IEEE Journal of Solid-State Circuits, IEEE, Vol. 24, No. 5, October 1989.
8. Haiyan Zhu, Wenhua Yang, Gil Engel, and Yong-Bin Kim. “A Two-Parameter Calibration Technique Tracking Temperature variations for Current Source Mismatch.” IEEE Transactions on Circuits and Systems – II: Express Briefs, IEEE, Vol. 64, No. 4, April 2017.
9. “Constant Switching for Signal Processing.” U.S. Patent US6842132 B2, January 2005.
10. Sungkyung Park, Gyudong Kim, Sin-Chong Park, and Wonchan Kim. “A Digital-to-Analog Converter Based on Differential-Quad Switching.” IEEE Journal of Solid-State Circuits, IEEE, Vol. 37, No. 10, October 2002.
11. Gil Engel, Shawn Kuo, and Steve Rose. “A 14-Bit 3 GHz/6 GHz Current-Steering RF DAC in 0.18 µm CMOS with 66 dB ACLR at 2.9 GHz.” 2012 IEEE International Solid-State Circuits Conference, IEEE, 2012.
12. Daniel Fague. “New RF DAC Broadens Software-Defined Radio Horizon.” Analog Dialogue, Vol. 50, No. 7, July 2016.
13. Patrick Pratt and Frank Kearney. “Ultrawideband Digital Predistortion (DPD): The Rewards (Power and Performance) and Challenges of Implementation in Cable Distribution Systems.” Analog Dialogue, Vol. 51, No. 07, July 2017.
Daniel E. Fague is the director of systems application engineering in the High Speed Products Group at Analog Devices. He received his B.S.E.E from Gonzaga University in 1989 and his M.S.E.E. from the University of California at Davis in 1991. He joined Analog Devices’ Wireless Handset Group in 1995; since joining the High Speed Products Group in 2011, Dan has focused on RF converter development. He holds seven patents and has published more than 30 articles and papers. He can be reached at [email protected]. Steven C. Rose is a staff design engineer in the High Speed Products Group at Analog Devices. He received his B.S.E.E. from the University of Michigan in 1999 and his M.S.E.E. from the University of California at Berkeley in 2002. He joined Analog Devices’ High Speed Conversion Products Group in 2002; since 2009, Steve has focused on the design of RF DACs. His email is [email protected].
Analog Devices
www.analog.com