Optical communication: The next defense & aerospace satellite frontier
StoryJune 14, 2023
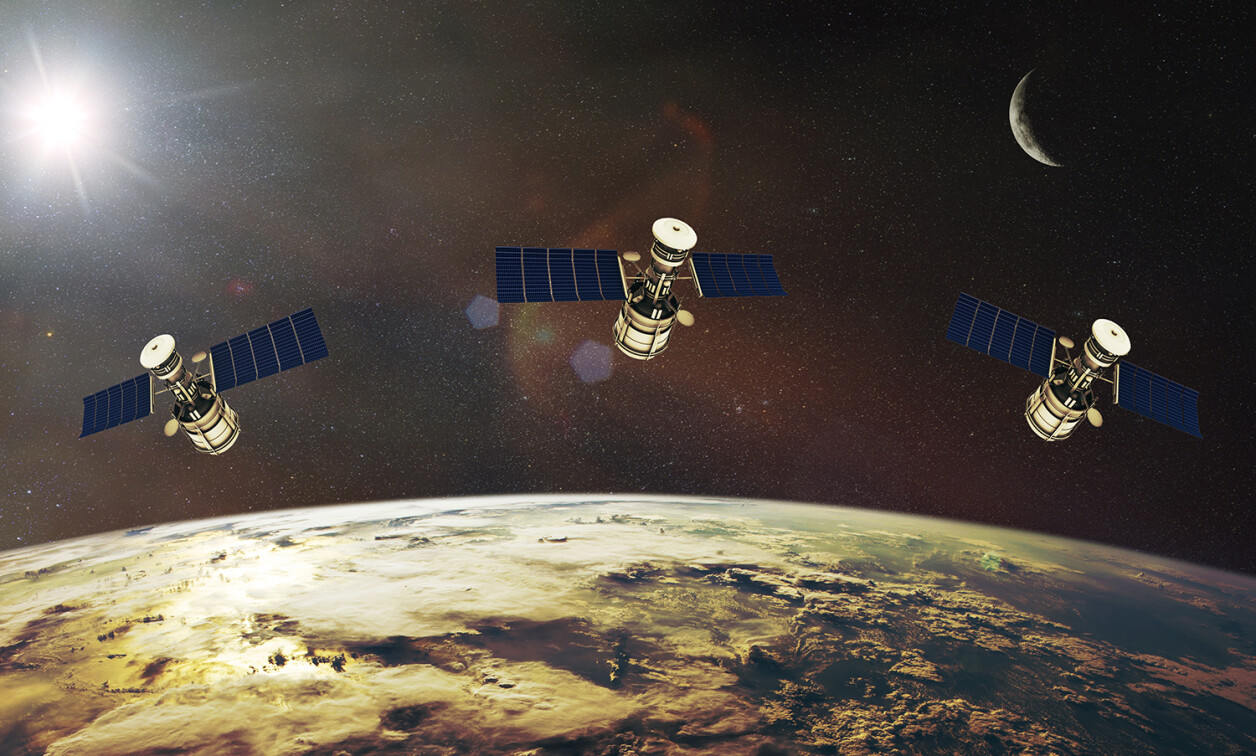
The space race is accelerating the need to quickly deploy new technologies and systems, prompting a move toward optical photonics communication systems that leverage commercial off-the-shelf (COTS) components.
Currently, the military relies on nonrobust satellite connections that lack sufficient bandwidth. The rollout of satellite networks in low earth orbit (LEO) eliminates these hurdles by lowering the barriers to entry. A new wave of LEO megaconstellations is emerging as a result, creating a growing opportunity for optical communication as the need for bandwidth grows exponentially. To take advantage of the benefits of optical communication, the easiest path for the satellite industry is to leverage commercial off-the-shelf (COTS) components. However, the risks and costs within the space industry are unique: Before a COTS component goes into orbit, it requires comprehensive testing to ensure it can reliably perform in space. From an engineering perspective, overcoming the harsh environment of space is a significant and mission-critical challenge.
The need to access space for navigation; communication; surveillance and reconnaissance; remote sensing; internet access; and space experimentation has never been greater and shows no sign of abating. Optical communication, which uses light to transmit information, provides the aerospace and defense industry with several advantages over traditional radio frequency (RF) communication. These benefits include increased bandwidth, higher frequencies, improved security, and the ability to transmit data without signal degradation.
Deploying optical links connects satellites and establishes a more resilient and secure network for flexible data transfer over longer distances. By leveraging optical links, the industry is hoping to eliminate the bandwidth and latency issues associated with traditional satellite systems.
A COTS approach to a harsh environment
Today, entrepreneurs and enterprises are fueling explosive growth and innovation, creating a “gold rush” to space. Technology advancements are the catalysts for much of this development, while the increase in competition has upended the space economy, leading costs to fall substantially. The following trends have lowered the barriers to entry, fueling rapid growth:
- reduced hardware costs
- reusable launch rockets
- densification of electronic components
- more standardization when connecting satellites to rockets
As the space rush intensifies, organizations are striving to be first to market by turning to COTS components widely deployed in other sectors. The adoption of COTS components reduces costs and speeds deployment; as the industry becomes more commercialized, the need to quickly integrate proven technologies will only continue to grow.
Taking advantage of components and technologies that are commonly used in terrestrial fiber-optic communication is an obvious path to minimize development costs in optical communicatios while optimizing performance. The benefits of this approach include the proven functionality and durability of both components and technologies for communications. Because the components are standards-based, designers also can use components from different manufacturers. Other advantages to already-proven parts include industrialized production, which typically enables rapid assembly and shorter lead times, as well as specifications and performance characteristics based on the volume of components and systems manufactured.
Now versus then
New satellite systems launching in LEO integrate COTS components to speed data transfer. To understand the growing need for COTS components, look at how satellite communications systems have changed over the last two decades. Figure 1 displays the entire communications chain of a simplified satellite communications system. The bent-pipe transceiver performs the following unique functions:
- receives the transmitted signal;
- filters and downconverts the signal to a lower RF frequency;
- amplifies and retransmits the same signal down to a receiver station.
[Figure 1 ǀ This graphic shows the entire communications chain of a simplified satellite communications system. (Image courtesy of Keysight.)]
The signal goes through amplification, filtering, and demodulation to pass the data into the network. However, these simplified systems have some limitations, including spotty connectivity. These performance issues pose challenges across industries, but especially in the defense sector. Military personnel must be able to access mission-critical insights on the battlefront or in remote locations at all times.
Figure 2 illustrates a more modern LEO satellite with internal-processing capabilities that enable it to change its function over time. In addition to the simple bent-pipe transceiver, modern systems can perform data processing within the satellite for data channelization, processing and even passing intelligence into the space network for optimized data transfer.
[Figure 2 ǀ This more modern LEO satellite boasts internal processing capabilities that enable it to change its function over time. (Image courtesy of Keysight.)]
Embedded capabilities are key to the success of networked mega-constellations. The addition of intersatellite links (ISL) enable the implementation of traffic routing in the satellite network. With the majority of satellite launches now comprising constellations or groups of satellites, these systems will be in constant contact with their counterparts on the ground. Many will also communicate with one another directly. The systems’ increasing sophistication signals a more mature era for ISLs and helps make a case for optical communication in space.
Figure 3 shows an optoelectronic satellite communications system. In the model, the laser becomes a localized reference for the ground-station telescope. The laser gets fed to an electro-optic modulator, and the data gets transmitted and encoded into the optical signal. The signal is then passed through the telescope optics to the satellite, which links its telescope and local references to an optoelectronic receiver. The satellite efficiently converts the data from optical to digital to enable processing before it converts the data back to optical and routing it in the relevant direction.
[Figure 3 ǀ In this example of an optoelectronic satellite communications system, the laser becomes a localized reference for the ground-station telescope. (Image courtesy of Keysight.)]
The case for optical communication in space
By using light to transmit data, optical communication offers specific advantages including achieving higher data rates. Occupied spectrum and interference with other systems are often communication hurdles when working with RF or microwave frequencies. These concerns are alleviated with the localized laser source and A-to-B communication associated with optical photonics. The benefit is that organizations can encode over wider bandwidths and obtain higher data rates.
Using a localized laser source is crucial to achieving the next frontier in satellite communications, as demand has recently begun to outpace the bandwidth provided by RF. The average transmission is currently tens or even hundreds of gigabits per second, and this is only expected to grow in tandem with new aerospace and defense innovations. In addition, optical communications are expected to play a critical role in supporting new Earth-observation technologies like synthetic aperture radar (SAR), a technology that enables organizations to send raw data to terrestrial applications in a highly secure manner.
Optical communication also supports increased security: SAR is just one of the use cases underscoring the importance of security. Optical communication’s tight, precise laser beam is much narrower than RF, meaning that the point-to-point communication of data is more secure. The laser beam protects optical links more effectively from eavesdropping, is more resistant to jamming and spoofing, and is better suited for confidential and military communication.
In addition, a laser’s physical makeup means that optical communication reduces energy dispersion in space. Unlike with RF, there is no need for a phased-array antenna for beam steering. Instead, optical communication uses fast-steering mirrors along with gimbals to ensure precision pointing with less energy dispersion.
The space network of tomorrow
As industries adopt optical communication, optical inter-satellite links (OISLs) will combine with intelligent routing to form a secure, robust network that rivals the terrestrial internet in speed and efficiency. Data remains in orbit, and artificial intelligence (AI) algorithms determine the most efficient ground station on Earth to deliver the information to the end user.
However, the industry must deploy new technologies and systems to realize this future. The most efficient and proven way to accomplish this is by using COTS components to accelerate deployment while keeping costs down. The use of COTS comes with one significant caveat, however. These technologies were not designed for space: a harsh environment replete with extreme temperature fluctuations, radiation exposure, and debris. Manufacturers need to ensure their COTS components can withstand these and other space-specific conditions without negatively affecting performance or reliability.
Simulate space conditions on earth
One of the best ways to determine whether COTS components can survive space conditions is by replicating space conditions on Earth and testing performance in the lab. Organizations can create simulated versions of systems and subsystems to ensure their COTS components can withstand any conditions in space. These emulations, known as digital twins, can significantly accelerate the development and decision-making processes. Digital twins enable designers to identify and address issues in the design phase rather than after the component is incorporated into a space system. Digital twins can also expose mission risks that might not be evident via traditional testing solutions and may also enable insight into how various COTS components will perform together in space conditions.
By bringing space into the lab and creating digital twins, COTS manufacturers can make better models, validate designs as they build the hardware, reduce the likelihood of failure in orbital demonstrations, and – ultimately – accelerate deployment. Looking at satellites specifically, power and the dynamic channel are two areas of operation that have a bearing on validating COTS optoelectronic systems. A satellite has no power source in space, and it must be self-sufficient once in orbit. Leakages or drains that exceed the satellite’s ability to generate and store power are tantamount to equipment failure. To test for power weaknesses in the lab, solar-array simulation can be performed to provide realistic power to the systems and subsystems, combined with power-analysis hardware that monitors the power distribution and current draw in an emulated environment.
For dynamic-channel emulation, it is critical to examine how Doppler, absorption, and scattering occur over time between communicating terminals – and also how these change based on orbits. For example, these factors may not be very important with ISLs as relative movement between satellites in the same orbit has minimal impact. However, linking with satellites in other orbits and to ground stations can impose higher Doppler and fading; users must test for variances to ensure they will not impede communications after launch.
It is also essential to test for launch vibration and forces, radiation damage, outgassing, space debris, and the components’ ability to withstand temperature extremes. Assessing performance across these areas and collecting the corresponding measurement data throughout simulation can give COTS manufacturers a solid foundation for in-orbit demonstration and – assuming all goes well – launch. A receiver optical sub-assembly (ROSA) may be used to convert an optical signal to an electrical one. A typical goal when testing this application is determining the wavelength-dependent responsivity of the photodiode and its polarization-dependent loss.
The future is COTS
The space gold rush is creating an array of possibilities, and organizations are racing to deploy new technologies and systems. Optical communication is just one path to transforming satellite systems. Using light to transmit data in space brings significant benefits, including higher data rates, improved security, and lower energy dispersion. As organizations build large satellite constellations, they are increasingly turning to COTS components to reduce costs and speed up deployment.
For manufacturers looking to harness COTS, the ability to evaluate if those components are space-ready is the pathway to participating in the boom. As the space industry becomes increasingly commercialized, the need to quickly integrate proven technologies that perform reliably in space will continue to grow. By emulating and evaluating on the ground, it can be determined whether COTS components are ready for the rigors of space.
Nancy Friedrich is an aerospace and defense industry solutions marketer at Keysight, specializing in areas ranging from space/satellite to EMSO, 5G, and military comms. Previously, Nancy was editor-in-chief of Microwaves & RF magazine.
Keysight https://www.keysight.com/us/en/home.html